Restoring the land to restore the sea

June 4, 2024 Benjamin Jones Chief Conservation Officer This year’s World Environment Day campaign focuses on land restoration, under the slogan “Our land. Our future. We are #GenerationRestoration.” Loss and degradation of coastal marine ecosystems, compromise the delivery of important ecosystem services to human society. Yet turning the tide on these losses and working towards a net gain in biodiversity is a challenge, not least because coastal marine ecosystems are exposed to threats occurring both in the ocean and on land. Land use change, through conversion of native terrestrial vegetation for agriculture, urbanization, and industry increases runoff and sedimentation, causing degradation of coastal ecosystems such as seagrass meadows. Swathes of evidence from across the globe reveal that anthropogenic activities from the land are some of the largest drivers of seagrass loss. For example, research from the Philippines has revealed that land use is more important than marine protection for tropical seagrass condition, and our own research has revealed the agricultural drivers of seagrass degradation across the British Isles. Yet for the most part, conservation prioritisation for coastal ecosystems is traditionally centred around protecting intact habitats from ocean-based stressors (e.g., fishing). If we are to conserve seagrass, we need to look beyond the ocean, and to the land. And this year’s World Environment Day is a key reminder of that. To conserve seagrass, should we be protecting habitat on land, protecting habitat in the ocean, restoring habitat on land, restoring habitat in the ocean, or a mixture of these actions? Answering this question is extremely difficult, not least when data is absent, histories of change are blurred, priorities for monitoring and management change and the nature and extent of threats to seagrass are unknown. In these instances, we believe that it is vital to understand which threats local stakeholders observe or perceive as being most persistent. Interweaving indigenous and local knowledge, and other expert witness knowledge as alternative data sources, is key. Our land. Our future. Seagrass meadows in the Wakatobi National Park (WNP), Indonesia are exploited for their rich fish and invertebrate communities – faunal communities that provide food security and livelihoods across the National Parks islands. Yet, with a growing population, the area of seagrass habitat is decreasing, and plant species composition and health is declining. Working with our local partner, FORKANI, and after a series of focus groups with local stakeholders, it seemed clear that the issue and threat they felt was most dominant, was sedimentation; the removal of mangroves and primarily forest areas had lessened the ability for land to absorb and store water. Built on indigenous and local knowledge, FORKANI, in collaboration with Project Seagrass, developed an incentives programme designed to provide fruit trees to farmers and landowners to facilitate stabilisation of river banks and reduce sediment deposition to the coast and at the same time improve the continually worsening problem of water storage. Now, over 5000 trees have been planted along riverbanks across the National Park by FORKANI, as well as school groups and government ministers. Photo: Nusi, a member of FORKANI, tends to trees in a nursery. Photo: Teenagers and school groups also took part in tree planting.
Want to host the 16th International Seagrass Biology Workshop?
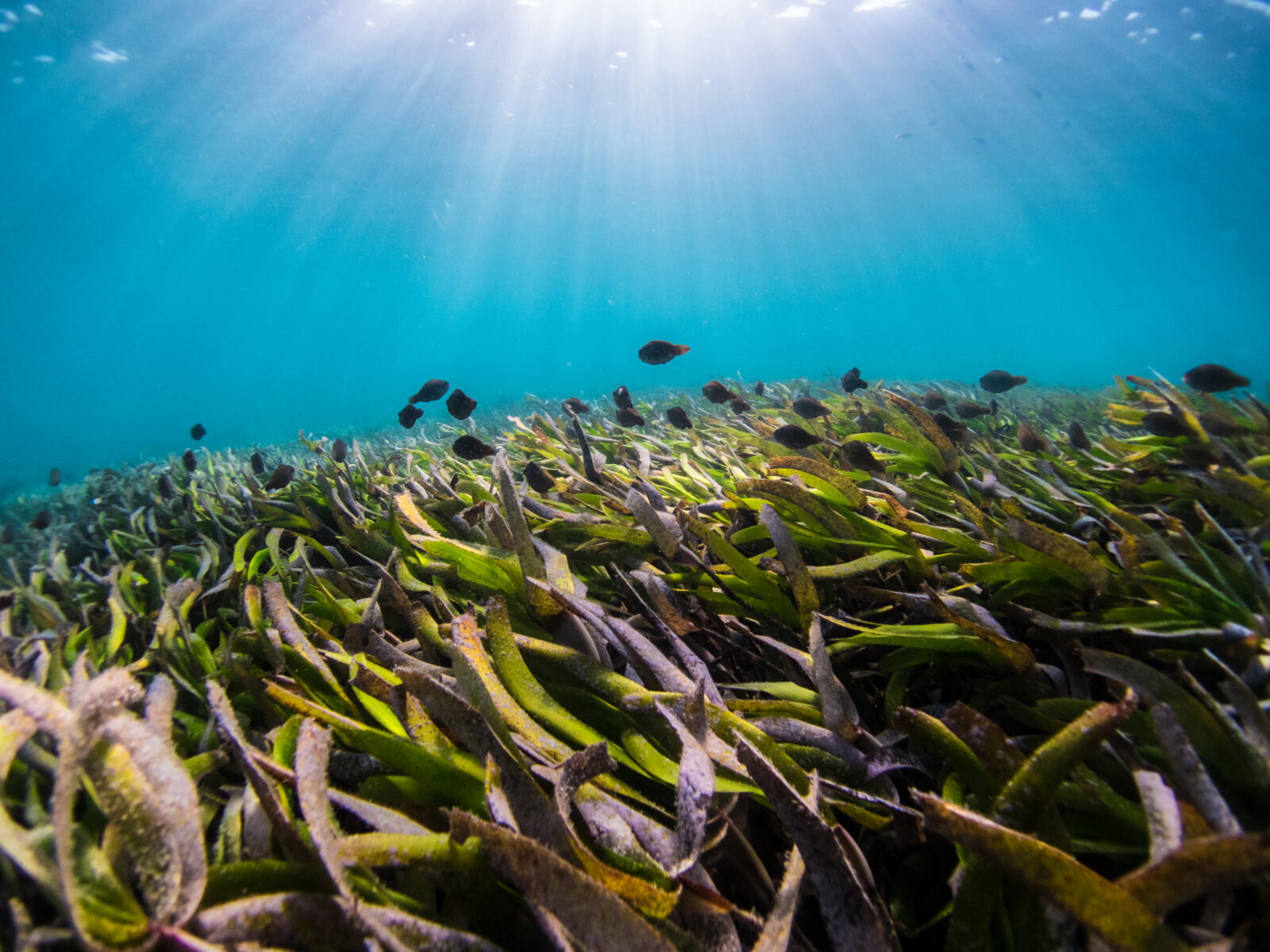
The World Seagrass Association Inc. invites expressions of interest from members and interested organisations/institutions who would like to host the 16th International Seagrass Biology Workshop (ISBW) in 2026. The International Seagrass Biology Workshop series is a meeting of research scientists, students and coastal environment managers focusing on global seagrass issues, improving seagrass knowledge, developing networks and advocating for seagrass protection/conservation. ISBWs are often 4-6 day events, made up of 2-3 days of talks (in a conference setting) and 1-2 days of workshops and often include an additional day for a field trip. Participants are generally around 100 individuals, however, this is highly dependent on the venue. The timing for ISBWs has mostly been late September, although the timing is dependent on what is most suitable for the hosting country (e.g. tides, holidays, etc). We would also like to encourage organisations/institutions in localities that have been underrepresented in past ISBWs to consider hosting this event to highlight the need for seagrass research and to expand our research networks. After the first official ISBW in 1993 it was decided that meetings were to be held at two year intervals, swapping between developed and developing nation locations. The 2024 meeting (ISBW15) will be held in Naples, Italy, from 17-21 June, in conjunction with the World Seagrass Conference (WSC2024) (https://www.isbw15.it/). Past ISBW venues include:ISBW1 (1993) Japan – KominatoISBW2 (1996) Australia – Rottnest IslandISBW3 (1998) The Philippines – Quezon City and BolinaoISBW4 (2000) France – Corsica!SBW5 (2002) Mexico – EnsenadaISBW6 (+Conference) (2004) Australia -Townsville and Magnetic islandISBW7 (2006) Africa – ZanzibarISBW8 (2008) Canada – Bamfield, Vancouver islandISBW9 (+Conference) (2010) Thailand – Phuket and Trang ProvinceISBW10 (2012) Brazil – BuziosISBW11 (2014) China – Sanya, Hainan ProvinceISBW12 (2016) United Kingdom – Nant Gwrtheyrn, WalesISBW13 (+Conference) (2018) SingaporeISBW14 (+Conference) (2022) USA – Annapolis, Maryland To read about the history of the ISBW series, seeColes, R., Short, F., Fortes, M. and Kuo, J (2014) Twenty years of seagrass networking and advancing seagrass science: The International Seagrass Biology Workshop Series. Pacific Conservation Biology 20(1): 8–16. (http://www.publish.csiro.au/nid/302/paper/PC140008.htm), andHind-Ozan, E.J., Jones, B.L., 2017. Seagrass science is growing: A report on the 12th International Seagrass Biology Workshop. Marine Pollution Bulletin.http://dx.doi.org/10.1016/j.marpolbul.2017.08.017 The International Seagrass Biology Workshop (ISBW) is an official activity of the World Seagrass Association Inc., and WSA Inc. plays a guiding role, and assists the Convener/Organising Committee when requested. Members or organisations/institutions who are interested in hosting the 16th International Seagrass Biology Workshop (ISBW) in 2026 should submit an Expression of Interest by email to Len McKenzie, Secretary, World Seagrass Association Inc., at wsa.secretary@gmail.com no later than March 01, 2024.Expressions of Interest should be no longer than 3 pages and include the following:• Name of Applicant• Applicant’s contact information including physical address and email address• Proposed Hosting Institution (add a brief description)• Country and proposed location for the event• Institutional Support• Tentative Venue Details (conference, workshop and field trips)• Preliminary Proposed Theme• Travel & Visa Considerations• Health & Safety Considerations• Diversity, Equity & Inclusivity ConsiderationsThe WSA may require more detailed information in future before a final decision. If you require additional information or any enquiries, please email wsa.secretary@gmail.com Previous Post January 15, 2024 ISBW press release updates World Seagrass Association
You asked, we listened. Our latest updates to SeagrassSpotter
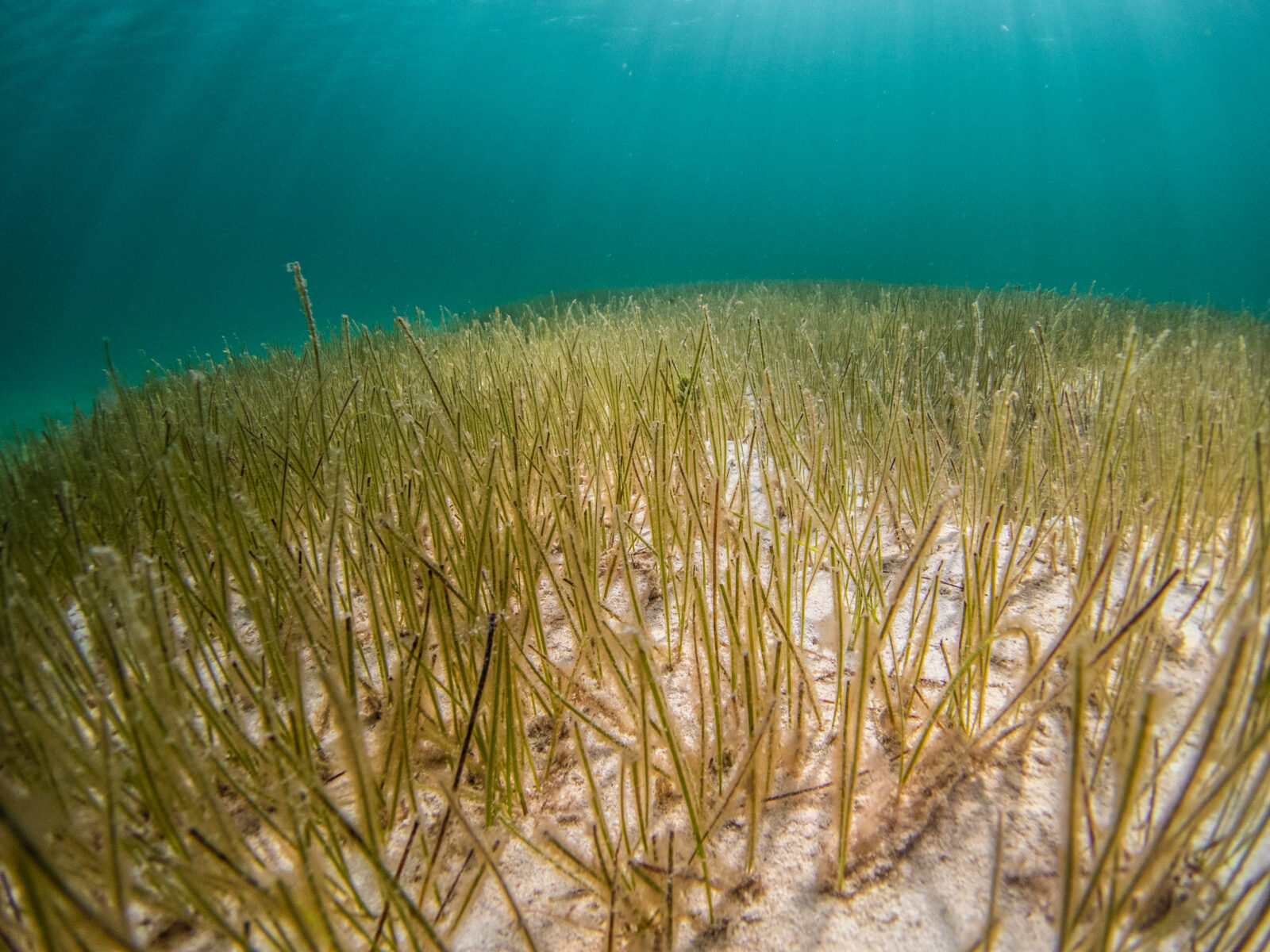
Things have been busy at Project Seagrass with lots of development on SeagrassSpotter. Since our last major update, we have been working to enhance the ways that SeagrassSpotter works for its users. We’re excited to share that our latest update includes many of the features you have been asking for, and a few more. One of the six main global challenges to seagrass conservation is that the status of many seagrass meadows is unknown, and up-to-date information on status and condition is essential for conservation. While we’re making great strides in using drone technology and satellite imaging for seagrass mapping, new technologies still depend on “ground-truth” data, which is where SeagrassSpotter comes in. This is how individuals can make lasting contributions to seagrass conservation. With this in mind and coupled with the requests you’ve made, we’ve released a new version of SeagrassSpotter. We’ve developed a completely new exploration and filter function, allowing you to select data from certain species, countries, and more. We’ve also made it easier for you to download SeagrassSpotter data and generate reports on the things that matter most. Finally, we’ve added a completely new upload function for absence data; places where seagrass isn’t found or used to be found but now isn’t – data that will help us and other transform habitat suitability modelling. Take a look at our feature recaps below to learn about each one in depth. Data exploration We understand the importance of being able to view the data you collect – you can see in real time how you’re contributing to the bigger picture. Data exploration is the first step of data analysis and is used to explore and visualize data to uncover insights from the start or identify areas or patterns to dig into more. Using interactive filtering, users can better understand the bigger picture and get to insights faster. You can filter data by any of the parameters that SeagrassSpotter collects (e.g., algae, fishing activity, flowering) and display this either in grid format or map format. Report generation In addition to our new explore tab, we’ve added new report generation features that allow you to download data that is filtered by any of the parameters that you select. Only want seagrass data from the Philippines? No problem! We understand the importance of being able to easily download data and have worked to remove barriers to this. All SeagrassSpotter data is free to access, and you don’t even need to sign up for an account. Open Access data will make conserving seagrass easier for everyone. Absence data It’s imperative that we know where seagrass is growing, but to advance seagrass restoration, it’s also important that we know where seagrass isn’t growing, and why. Whether it’s been lost, or simply was never found there in the past, our new absence data function allows you to add data on other habitats and substrates such as mangroves, coral, seaweeds, rocks, sand and mud. This will make habitat suitability modelling easier and more efficient, while also adding greater ground truthing needs for remote sensing. New languages We understand that for SeagrassSpotter to be a global tool, it needs to be accessible to all, no matter what language you speak. Over the past few years, we’ve slowly been adding additional languages to increase usability in many of the countries we work. Alongside this, we’re developing an app within SeagrassSpotter called SpotLang that provides a means for anybody to help curate new languages with us. As it currently stands, SeagrassSpotter is available in nine languages; English, Welsh, French, Portuguese, Swahili, Thai, Bahasa Indonesia, Bahasa Malay and Tetun. If you are interested in contributing to new languages, please get in touch.
Seagrass production around artificial reefs is resistant to human stressors
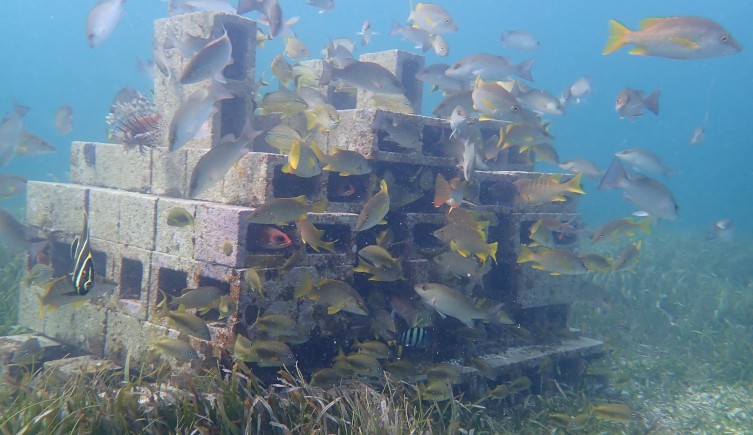
Artificial reefs might help to restore the ocean’s ability to fight against climate change. The reefs boost the productivity of seagrass meadows by attracting fish, which can improve the ability of these habitats to lock up more carbon dioxide beneath the waves. Breeze blocks placed in one of the ocean’s most endangered habitats provide an unexpected lift for fish. Seagrass meadows are found across the world, reaching from the tropics up into the lower reaches of the Arctic circle. They are incredibly valuable habitats, providing a nursery for young fish as well as sucking vast quantities of carbon dioxide from the atmosphere. However, with an area of seagrass the size of a football pitch being lost every 30 minutes, it’s more important than ever to find out how to turn things around. A new study in the Caribbean has shown that artificial reefs can help to bolster their growth in the tropics, even as threats such as fishing and nutrient pollution continue. Dr Jacob Allgeier, a co-author of the paper, says, ‘By attracting fish, whose faeces provide concentrated nutrients for the seagrass, the artificial reefs increase the primary production of the entire ecosystem.’ ‘We are now investigating how this cascades up the food web. The new energy has to go somewhere, so we are quantifying how it affects invertebrates and fish with our evidence suggesting that it is fuelling increases in both.’ The findings of the study were published in Proceedings of the Royal Society B. Artificial reefs and seagrass One of the biggest issues affecting seagrass is nutrient pollution, often from the release of human sewage. While the influx of nutrients can initially boost the growth of the meadows, it also promotes the growth of algae which reduces the amount of sunlight getting to the seagrass and harms it in the long run. Alongside fishing which causes levels of the fish faeces that fertilise the meadows to drop, it was thought that the combination of these two issues might work in unexpected ways to hinder the growth of seagrass. But the current study has revealed some surprising results. It has found that the productivity of seagrass in both disturbed and undisturbed meadows was increased by the presence of an artificial reef, while algae didn’t actually seem to pose an issue, even in areas where nutrient pollution was high. Mona Andskog, the PhD student who led the research, explains, ‘Artificial reefs built in seagrass create a positive feedback loop. They attract fish that use the reefs for shelter which, in turn, supply new nutrients from their faeces that fertilise the seagrass around the reef.’ ‘This increased primary production can increase invertebrate production by providing more food and shelter for invertebrates, which in turn provide more food for fishes.’ Experiments in Haiti, at some of the most fished sites included in the study, also showed that the artificial reefs were providing additional benefits to the fish. Large numbers of small fish were found at the site because of the difficulty in using nets around the reef, meaning that the overall biomass of fish was at times larger than in unfished areas measured elsewhere in the study. While artificial reefs present a promising option for tropical seagrasses, they’re likely to have a much more limited impact on temperate meadows. These waters already tend to have higher nutrient levels, meaning that any contribution the reef would made to overall growth would be small. The scientists now hope to explore how the placing of artificial reefs can affect seagrass ecosystems, as well as expanding their research to the Dominican Republic. ‘We will be testing how different configurations of artificial reef clusters can affect the production and fish community composition,’ Jacob says. ‘This includes the number of artificial reefs in each cluster, as well as their arrangement.’ ‘As with this research, we hope to simultaneously use the reefs to test fundamental questions about production in these highly impacted ecosystems as well as optimising the positive feedback that is initiated by the artificial reefs.’ More information: Mona A. Andskog et al, Seagrass production around artificial reefs is resistant to human stressors, Proceedings of the Royal Society B: Biological Sciences (2023). DOI: 10.1098/rspb.2023.0803 This story is republished courtesy of Natural History Museum. Read the original story here.
How eelgrass spread around the world
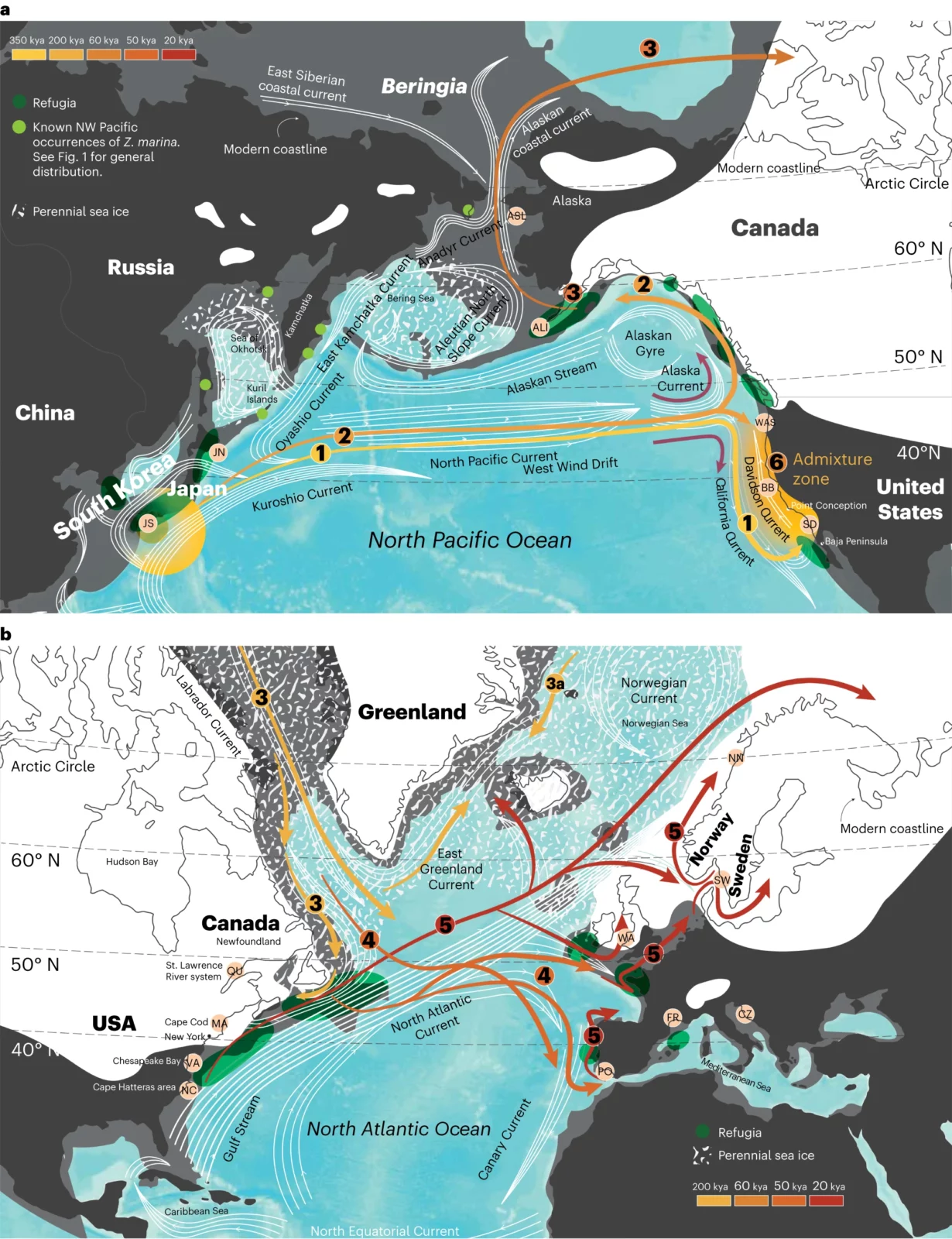
Seagrasses evolved from freshwater plants and use sunlight and carbon dioxide (CO2) for photosynthesis and are able to thrive in depths down to 50 meters. In contrast to algae, they possess roots and rhizomes that grow in sandy to muddy sediments. The grass-like, leaf-shoots produce flowers and complete their life cycle entirely underwater. Seeds are negatively buoyant but seed-bearing shoots can raft, thus greatly enhancing dispersal distances at oceanic scale. As a foundational species, eelgrass (Zostera marina) provides critical shallow-water habitats for diverse biotas and also provides numerous ecosystem services including carbon uptake. Seagrasses have recently been recognized as one of the important nature-based contributions to store carbon in the ocean. The sediment below seagrass meadows can sequester between 30 and 50 times more carbon annually that the roots of forests on land. Unfortunately, the continuing loss of seagrass beds worldwide—including eelgrass—is of acute concern. An international group of researchers, including Richard Unsworth and coordinated by Professor Thorsten Reusch, Head of the Research Division Marine Ecology at GEOMAR Helmholtz Centre for Ocean Research Kiel, used complete nuclear and chloroplast genomes from 200 individuals and 16 locations to reconstruct and date the colonisation history of the eelgrass Zostera marina from its origin in the Northwest Pacific Ocean to the Pacific, Atlantic and the Mediterranean. The findings described in an article and a Research Briefing published in Nature Plants beg the question, “How well will eelgrass adapt to our new, rapidly changing climate?” Using a phylogenomic approach the scientists were able to determine that Z. marina first arose in the Japanese Archipelago region and then crossed the Pacific from west to east in at least two colonisation events, probably supported by the North Pacific Current. The scientists then applied two DNA “molecular clocks”—one based on the nuclear genome and one based on the chloroplast genome—to deduce the time when eelgrass populations diverged into new ones. The DNA mutation rate was calculated and calibrated against an ancient, whole genome duplication that occurred in eelgrass. Both nuclear and chloroplast genomes revealed that eelgrass dispersed to the Atlantic through the Canadian Arctic about 243 thousand years ago. This arrival is far more recent than expected—thousands of years versus millions of years, as is the case with most Atlantic immigrant species during the Great Arctic Exchange some 3.5 million years ago. Reusch explains, “We thus have to assume that there were no eelgrass-based ecosystems—hotspots of biodiversity and carbon storage—in the Atlantic before that time. Recency was also mirrored in an analysis of the associated faunal community, which features many fewer specialized animals in the Atlantic as compared to the Pacific eelgrass meadows. This suggests that there was less time for animal-plant co-evolution to occur.” Mediterranean populations were founded from the Atlantic about 44 thousand years ago and survived the Last Glacial Maximum. By contrast, today’s populations found along the western and eastern Atlantic shores only (re)expanded from refugia after the Last Glacial Maximum, about 19 thousand years ago—and mainly from the American east coast with help from the Gulf Stream. In addition, the researchers further confirmed the huge difference in genomic diversity between the Pacific and Atlantic, including latitudinal gradients of reduced genetic diversity in northern populations. “Both Atlantic compared to Pacific populations, and northern versus southern ones are less diverse on a genetic level than their ancestors by a factor of 35 among the most and least diverse one,” said postdoctoral scientist Dr. Lei Yu, first author of the publication, which was a chapter in his doctoral thesis. “This is due to bottlenecks arising from past ice ages, which raises concerns as to how well Atlantic eelgrass, will be able to adapt to climate change and other environmental stressors based on its genetic capacity.” “Warming oceans have already caused losses of seagrass meadows at the southern range limits, in particular North Carolina and southern Portugal. In addition, heat waves have also caused losses in shallow waters in some the northern parts of the distribution,” noted Reusch. “This is not good news because seagrass meadows form diverse and productive ecosystems, and no other species is able to take on the role of eelgrass if meadows cannot persist under future conditions.” “One possibility for restoration might be to borrow some genetic diversity from Pacific eelgrass to fortify diversity in the Atlantic. Our next step is to interrogate the eelgrass pangenome. A new reference genome from Pacific eelgrass is currently under development and should tell us more about the adaptive ecotypic capacity across its global range of habitats,” said Prof. Jeanine Olsen, emeritus professor from the University of Groningen who initiated the study and coordinated the work between the Joint Genome Institute (JGI) and the research team. More information: Yu, L. etal, Ocean current patterns drive the worldwide colonization of eelgrass (Zostera marina), Nature Plants (2023). DOI: 10.1038/s41477-023-01464-3 Story provided by Helmholtz Association of German Research Centres
Green sea turtles have traveled to the same seagrass to eat for 3,000 years
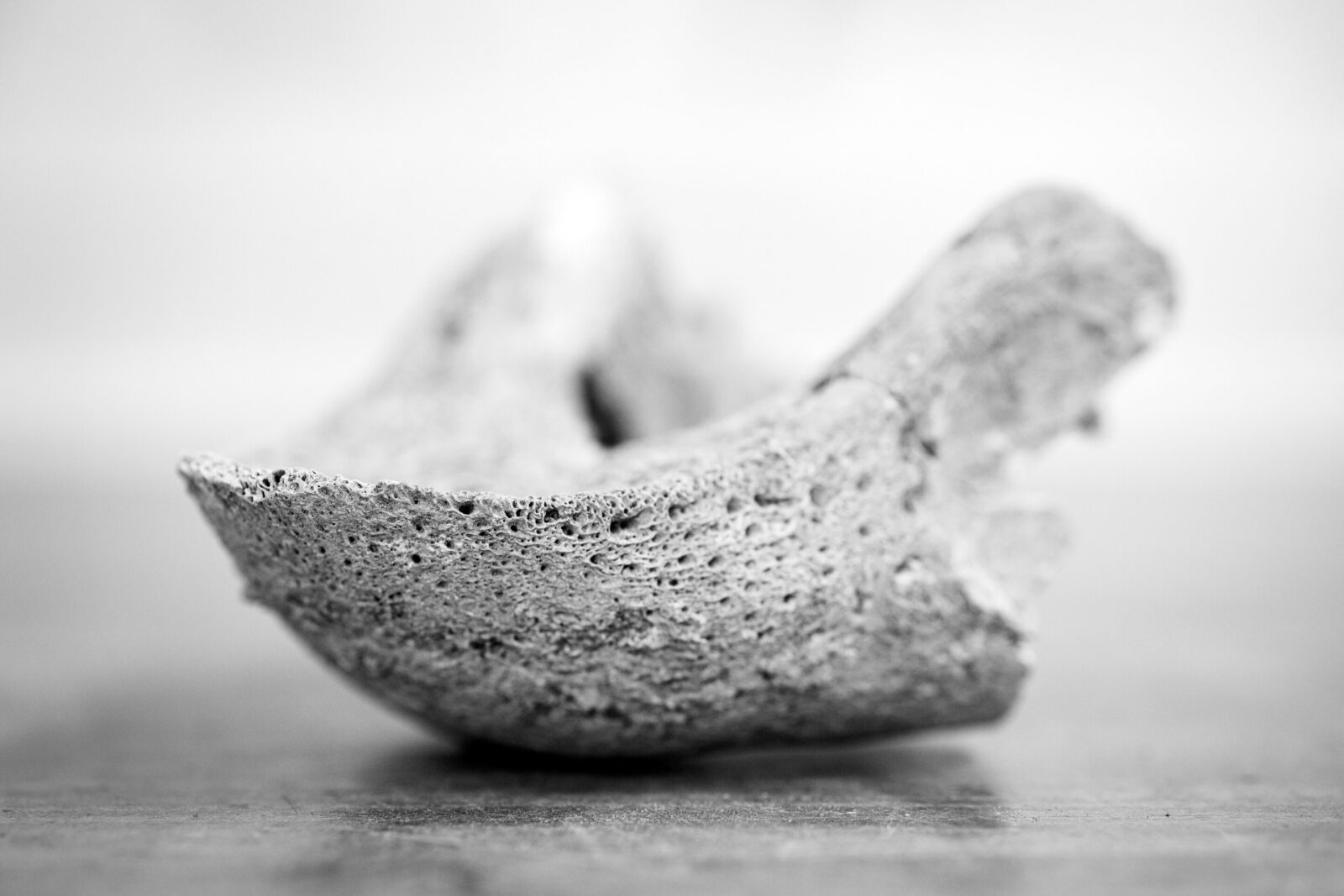
For approximately 3,000 years, generations of green sea turtles have returned to the same seagrass meadows to eat. This was discovered by Willemien de Kock, a historical ecologist at the University of Groningen, by combining modern data with archaeological findings. Sea turtles migrate between specific breeding places and eating places throughout their lives–this much was known. But the fact that this stretches over many generations highlights the importance of protecting seagrass meadows along the coasts of North Africa. The results were published in PNAS on July 17. When young green sea turtles hatch, their parents have already left for a long journey. The little turtles clumsily make their way off the beach into the ocean and, not yet able to navigate the long migration of their parents, float around for years. During this time, they are not very picky eaters, omnivores even. Then, at about five years of age, they swim to the same area where their parents went, to eat a herbivore’s diet of seagrass. Along the coasts of the eastern Mediterranean Sea, volunteers are active to protect the nests of the endangered green sea turtles. However, as Willemien de Kock explains, “We currently spend a lot of effort protecting the babies but not the place where they spend most of their time: the seagrass meadows.” And crucially, these seagrass meadows are suffering from the effects of the climate crisis. Analyzing sea turtle bones In the attic of the Groningen Institute of Archaeology at the University of Groningen, De Kock had access to boxes full of sea turtle remains from archaeological sites in the Mediterranean Sea area. The excavations were already done by her supervisor, Dr. Canan Çakırlar. “All I had to do was dig in some boxes,” De Kock says. By analyzing the bones, De Kock was able to distinguish two species within the collection of bones: the green sea turtle and the loggerhead turtle. De Kock was also able to identify what the sea turtles had been eating. This relied on a substance called bone collagen. By inspecting the bone collagen with a mass spectrometer, De Kock could detect what kind of plants the sea turtles must have eaten. “For instance,” De Kock explains, “one plant might contain more of the lighter carbon-12 than another plant, which contains more of the heavier carbon-13. Because carbon does not change when it is digested, we can detect what ratio of carbon is present in the bones and infer the diet from that.” Combining old and new Modern satellite tracking data from the University of Exeter then provided De Kock with information on the current traveling routes and destinations of sea turtles. Researchers from Exeter had also been taking tiny samples of sea turtles’ skins, which revealed similar dietary information as De Kock found in bones. De Kock was, therefore, able to draw conclusions, connecting diets of millennia ago to specific locations. She found that for approximately 3,000 years, generations of green sea turtles have been feeding on sea grass meadows along the coasts of Egypt and West Libya. The results for loggerhead turtles were less specific because they had a more varied diet. So, why is it relevant to know the eating habits of a species over many past generations? Because we collectively suffer from the shifting baseline syndrome: slow changes in a larger system, such as an animal population, go unnoticed because each generation of researchers redefines what the natural state was, as they saw it at the start of their careers. “Even long-term data goes back only about 100 years,” says De Kock. “But tracing back further in time using archaeological data allows us to better see human-induced effects on the environment. And it allows us to predict, a bit.” In fact, recent models have shown a high risk of widespread loss of seagrass in precisely these spots where green sea turtles have been going for millennia. This could be detrimental to the green sea turtle, precisely because of its high fidelity to these places. More information: de Kock, Willemien, Threatened North African seagrass meadows have supported green turtle populations for millennia, Proceedings of the National Academy of Sciences(2023). DOI: 10.1073/pnas.2220747120 Story provided by University of Groningen
Caribbean seagrasses provide services worth $255B annually, including vast carbon storage
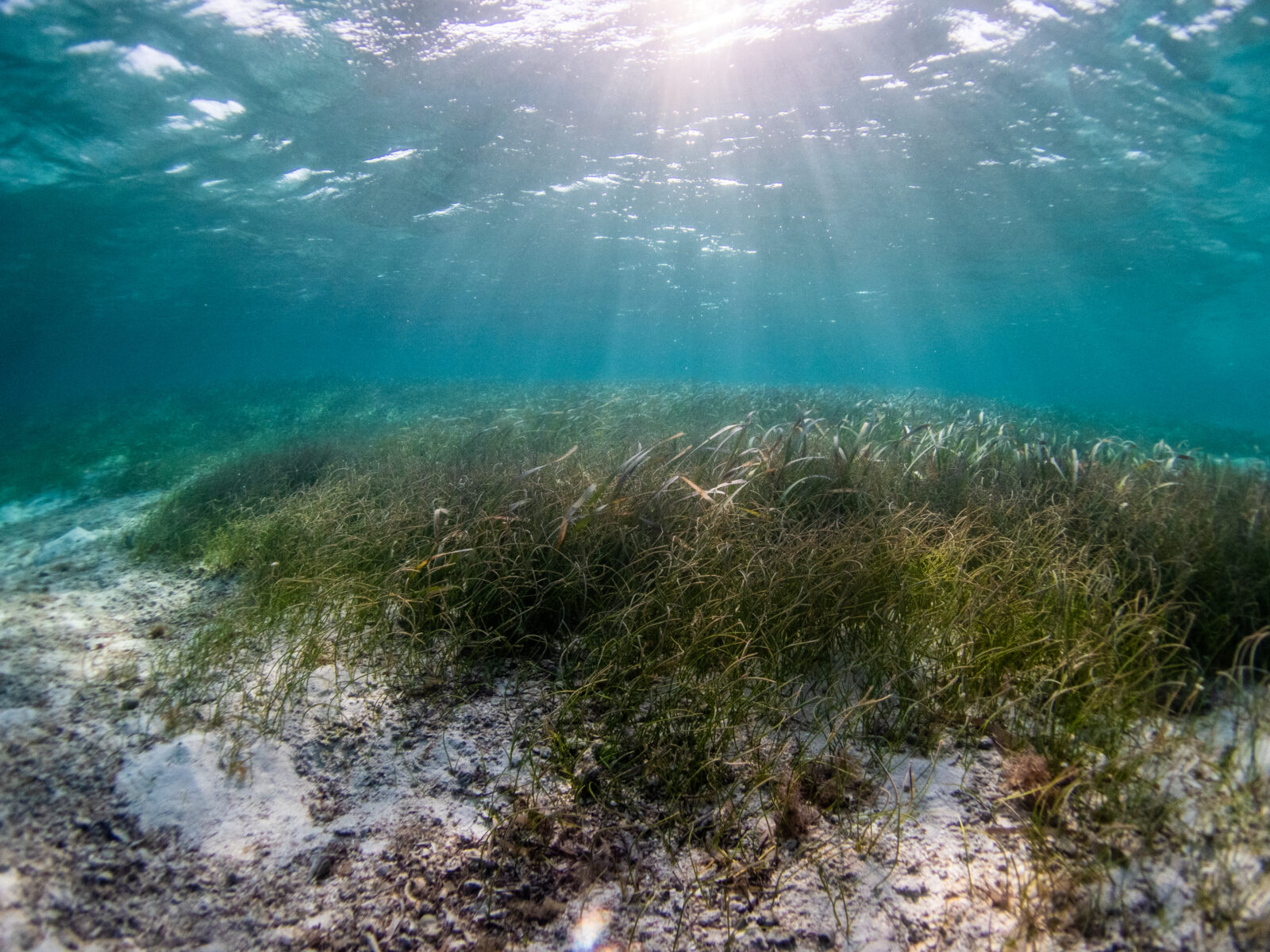
Discussions of valuable but threatened ocean ecosystems often focus on coral reefs or coastal mangrove forests. Seagrass meadows get a lot less attention, even though they provide wide-ranging services to society and store lots of climate-warming carbon. But the findings of a new University of Michigan-led study show that seagrass ecosystems deserve to be at the forefront of the global conservation agenda, according to the authors. It’s the first study to put a dollar value on the many services—from storm protection to fish habitat to carbon storage—provided by seagrasses across the Caribbean, and the numbers are impressive. Using newly available satellite data, the researchers estimate that the Caribbean holds up to half the world’s seagrass meadows by surface area, and it contains about one-third of the carbon stored in seagrasses worldwide. They calculated that Caribbean seagrasses provide about $255 billion in services to society annually, including $88.3 billion in carbon storage. In the Bahamas alone, the ecosystem services provided by seagrasses are valued at more than 15 times the country’s 2020 gross domestic product, according to the study published online June 21 in the journal Biology Letters. “Our study is the first to show that seagrass beds in the Caribbean are of global importance in their areal extent, in the amount of carbon they store, and in the value of the economic services they provide to society,” said study lead author Bridget Shayka, a doctoral student in the U-M Department of Ecology and Evolutionary Biology. “The findings underscore the importance of conserving and protecting these highly threatened and globally important ecosystems, which are critical allies in the fight against climate change.” One way to prioritize seagrass conservation would be to include those verdant undersea meadows in global carbon markets through projects that minimize loss, increase areal extent or restore degraded beds. The idea of selling “blue carbon” offset credits, which monetize carbon stored in coastal and marine ecosystems, is gaining traction for several reasons. For one, many island nations that have already been impacted by climate change—through increasingly intense hurricanes or rising sea levels, for example—have large areas of valuable coastal ecosystems that store carbon and that provide other services to society. Blue carbon (the name refers specifically to carbon stored in coastal and open-ocean ecosystems while “green carbon” refers more broadly to carbon stored in all natural ecosystems) offset credits could be a way for wealthier countries to compensate for their contribution to human-caused climate change while at the same time benefiting the economies of impacted countries and helping to conserve coastal ecosystems, which are among the most impaired in the world. Threats to seagrass meadows include coastal development, chemical pollution, recreation, shipping and climate change. “Because seagrass ecosystems are both highly important for carbon storage and sequestration, and are highly degraded globally, they represent an important burgeoning market for blue carbon,” said marine ecologist and study senior author Jacob Allgeier, an associate professor in the U-M Department of Ecology and Evolutionary Biology. “Yet, to date, a fundamental impediment to both evaluating seagrass and promoting it in the blue carbon market has been the lack of thorough seagrass distribution data.” For their study, the U-M-led team used newly available seagrass distribution data collected by the PlanetScope constellation of small DOVE satellites. They classified Caribbean seagrass ecosystems as either sparse or dense and estimated the amount of carbon in plants and sediments using data from Thalassia testudinum, the dominant seagrass species in the region. The researchers then calculated a conservative economic value for the total ecosystem services provided by seagrasses in the Caribbean and for the stored carbon, using previously published estimates for the value of services including food production, nursery habitat for fishes and invertebrates, recreation and carbon storage. Grouper, queen conch and lobster are among the commercially harvested animals that rely on Caribbean seagrass. Green sea turtles, tiger sharks and manatees also depend on it. To estimate the dollar value of the carbon stored in Caribbean seagrass beds, the researchers used $18 per metric ton of carbon dioxide equivalents, borrowed from California’s cap and trade program. In addition to Caribbean-wide estimates, the researchers calculated values for individual countries in the region: The Bahamas has the largest share of Caribbean seagrass (61%), providing total ecosystem services valued at $156 billion annually, including $54 billion in carbon storage. Cuba ranks second in areal seagrass coverage (33% of the Caribbean total), with a value of $84.6 billion per year for all ecosystem services, including $29.3 billion for carbon storage. The dollar value of the carbon in seagrasses around Cuba is equivalent to 27% of the country’s 2020 GDP. “Importantly, the degradation of seagrass beds often leads to erosion and sediment resuspension, which can create a positive feedback of increased seagrass loss and the release of C stored in sediments,” the authors wrote. “Blue carbon finance thus represents a potential mechanism by which the global community can invest in conserving and protecting these vital ecosystems.” More than 60 species of seagrasses grow in shallow coastal waters around the world. They evolved from land plants that recolonized the oceans 70 to 100 million years ago. In a separate paper accepted for publication in the journal Proceedings of the Royal Society, Allgeier and colleagues show that the construction of artificial reefs in the Caribbean can help protect seagrass ecosystems from human impacts, including nutrient pollution and overfishing. Seagrasses use photosynthesis to pull carbon dioxide from the atmosphere, then store the carbon in plant tissues. The seagrasses are quickly inundated by sediments, slowing decomposition. As a result, more than 90% of the carbon stored in seagrass beds is in the top meter of sediment. Caribbean seagrasses and associated sediments store an estimated 1.3 billion metric tons of carbon, according to the new study. That’s a big number, but it’s just 1.09% of the carbon contained in above- and below-ground woody biomass in the Amazon, and just 1.12% of the carbon in the biomass and soils of the world’s temperate forests, according to the new
Study reveals widgeongrass has replaced eelgrass as the dominant seagrass species in Chesapeake Bay
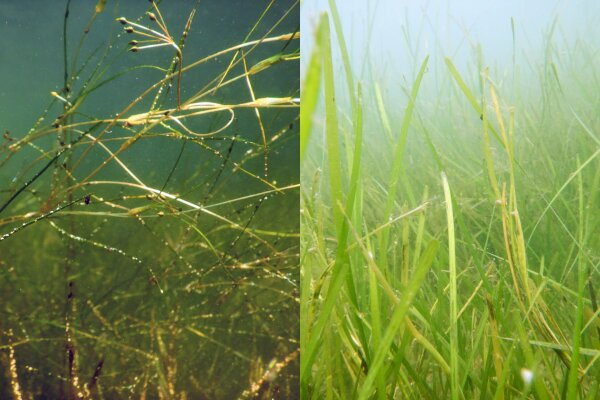
Mangroves are growing in areas historically dominated by salt marshes and oyster reefs. Invasive pacific oysters are replacing native blue mussels in the Wadden Sea. Macroalgae are exhibiting dominance over hard corals in the Caribbean and Indo-Pacific. Climate change-driven shifts in dominant, habitat-forming species such as these can have significant implications for conservation, and this is a phenomenon that seems to be particularly prevalent for seagrass systems around the world. In research published today in the Proceedings of the National Academy of Sciences, VIMS researchers and their collaborators evaluate the causes and consequences of a new dominant seagrass species rising in the Chesapeake Bay, demonstrating both new threats and management opportunities. For this study, the authors combined 38 years of data on nutrient and sediment pollution from runoff, temperature, plankton blooms, and river flow with aerial seagrass surveys to describe the causes and consequences of shifting seagrass foundation species across 26,000 hectares of habitat in Chesapeake Bay. Demonstrated shifts in seagrass create faster recovery but larger die-offs across Chesapeake Bay Marine heatwaves and poor water clarity in Chesapeake Bay over the last few decades have cut the area occupied by the previously dominant seagrass, eelgrass, in half. At the same time, successfully implemented nutrient reductions throughout the bay have encouraged the rapid expansion of another seagrass, the cosmopolitan widgeongrass. Confined to a fringing area of shallow brackish waters until the mid 1990s, widgeongrass has now replaced eelgrass as the most abundant seagrass in Chesapeake Bay by expanding over 150% due to both high temperature-tolerance and long-lasting seeds that allow for rapid recovery after disturbance. “Widgeongrass’ recovery and expansion ability is so strong,” explains lead author Hensel, “that ideal widgeongrass conditions have fueled two record-setting peaks for Chesapeake Bay seagrass cover. In fact, much of the nearly 300% increase in Bay plants since the mid 1990s has been widgeongrass expansion into areas that eelgrass has vacated.” However, some negative consequences from the shift have concerned habitat managers. “We’ve seen periods of rapid widgeongrass expansion and retraction for decades now, far beyond what we’ve documented for eelgrass,” says Landry, co-author and leader of the Chesapeake Bay Program’s Submerged Aquatic Vegetation (SAV) Workgroup. “But now that widgeongrass has become so widespread in the bay, its fluctuating abundance can have a big impact on our overall acreage trends and on our Bay-wide restoration goal attainment. That’s the management concern. The ecological concern is the impact those fluctuations are having on the animals that have grown to depend on widgeongrass for habitat in the absence of eelgrass.” The causes of these fluctuations have been difficult to understand because widgeongrass and eelgrass appear to respond differently to climate change and nutrient pollution stressors. Yet when the authors examined long-term, large-scale data on climate stressors and watershed pollution from agriculture and development in tandem with year-to-year aerial survey imagery of seagrass meadows, they recognized an important shift in the dominant climate stressor for Chesapeake Bay seagrass: while widgeongrass is resistant to heatwaves and high temperatures, it is highly vulnerable to periods in spring when high rains can bring huge influxes of nutrient- and sediment-loaded water into the bay, reducing water clarity. “This study is an important step forward in building our knowledge of human-ecosystem interactions along the coast and how they are changing over time,” says author Lefcheck. “Our past work showed a record-setting resurgence of underwater grasses in response to nutrient management, but now we are seeing that the story is vastly more complex and in fact, is still being written. Understanding, adapting to, and communicating this shifting narrative is a challenge, but not an insurmountable one by any stretch.” Conserving species with different needs simultaneously is necessary, complex The team’s modeling contributes to a greater understanding of both human and climate drivers of annual changes in widgeongrass and highlights a crucial difference in management compared to an eelgrass-dominated bay. “Heatwave stress is uncontrollable on a local and regional level,” explains Patrick, Director of the SAV Monitoring Program at VIMS, “but managing the amount of nutrients that enter the bay from the watershed during a rainy spring is something that we can actually control.” This study underscores that, because species differ in their traits and stressor sensitivities, managing for conservation of living habitats requires more community- or species-focused research, monitoring, and actions under climate change. Climate change is shifting the landscape of species composition, creating new winners in these novel environments, and management needs to shift alongside them. Detailed monitoring data allow agencies to focus on each species and encourages them to manage for community or individual habitat requirements, adapting strategies as species composition changes. This study also demonstrates pitfalls that can occur if habitat forming species are lumped together into single “stocks,” such as “hectares of seagrass” or “acres of marsh.” In fact, differences between the seagrass species in this study explain many of the shifts in Chesapeake Bay seagrass meadow dynamics. “Widgeongrass has shorter, thinner blades than eelgrass,” says Hensel, “which makes it more vulnerable to springtime run-off events because sunlight can’t reach the short blades through the clouded, nutrient-loaded water. Also, widgeongrass’ shallower root system may not sequester carbon as well and its tendency to wildly fluctuate in cover means that it may not provide consistent habitat for key seagrass-dependent species like Blue Crabs and Black Sea Bass.” The authors call for a parallel shift in coastal monitoring and evaluation of management successes and failures, using the Chesapeake Bay as an example. They cite the necessity of long-term monitoring programs with coordinated and standardized on-the-ground and detail-oriented surveys, as well as the importance of community or species-specific recovery goals across coastlines where multiple seagrass species co-occur and respond to climate differently. “This is a compelling example of the how climate change is unfolding across the globe,” said Patrick. “As regional climates shift, the emergence of novel ecosystems is fundamentally challenging everything we think we know from analysis of historical data. The rules governing the dynamics of the world’s ecosystems are changing and
What causes decline of tropical seagrass meadows?

Seagrass, a group of aquatic angiosperms, grows in shallow waters in the coastal sea and contributes most of the primary production while participating in many important ecological processes. Heat stress threatens the survival of seagrass, but its damage mechanisms are unclear. Recently, a research team led by Prof. Liu Jianguo from the Institute of Oceanology of the Chinese Academy of Sciences (IOCAS) primarily explained the physiological and biochemical mechanisms underlying the decline of tropical seagrass meadows caused by heat stress combined with high light at different functional levels. The study was published in Marine Pollution Bulletin. During the lowest tide, intertidal seagrass is frequently faced with the combined stress of exposure to air, direct sunlight, and high temperature, which may have a strongly negative impact on the survival of seagrass, especially in the context of global warming. The researchers found that the largest tropical seagrass Enhalus acoroides can withstand heat below 39°C in the dark. However, under high light, the tolerance to heat stress is greatly reduced. The combined effect of short-term exposure to heat and high light stress destroyed the photosystem II (PSII) and destroyed key components of the photosynthetic system in seagrass leaves. Moreover, high light combined with heat stress caused severe oxidative stress in the seagrass, which led to irreversible damage to the seagrass. These results clearly suggest that heat stress coupled with high light, may be an important cause for the decline of E. acoroides meadows. “Our study reveals that ocean warming, especially when coupled with high light, exacerbates the decline of seagrass meadows and affects the ecological function of intertidal seagrass meadows,” said Dr. Zhang Mengjie, first author of the study. “This serves as a warning that the effects of global warming on seagrass meadows will be even worse than expected,” said Prof. Liu, corresponding author of the study. More information: Mengjie Zhang et al, Heat stress, especially when coupled with high light, accelerates the decline of tropical seagrass (Enhalus acoroides) meadows, Marine Pollution Bulletin (2023). DOI: 10.1016/j.marpolbul.2023.115043
Fauna return rapidly in planted seagrass meadows, study shows
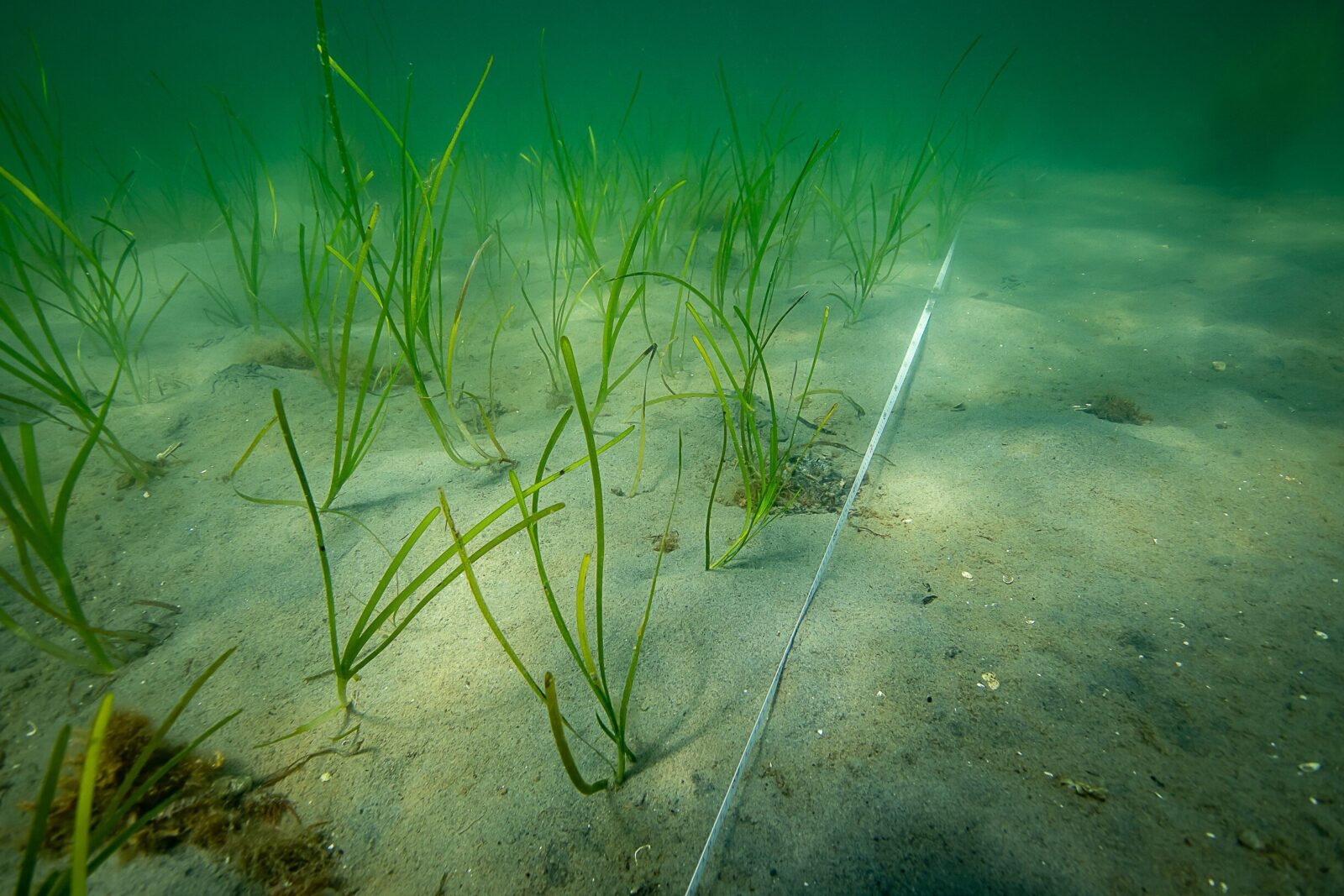
A study of eelgrass meadows planted by researchers from the University of Gothenburg shows that fauna return rapidly once the eelgrass has started to grow. Already after the second summer, the biodiversity in the planted meadow was almost the same as in old established eelgrass meadows. Eelgrass meadows have declined heavily in southern Bohus county in recent decades and in many places have disappeared altogether. Researchers at the University of Gothenburg have been working on the restoration of eelgrass meadows for twelve years. These meadows are important for biodiversity, as the eelgrass serves as habitat or nursery for young cod, crabs and shrimps for example. In a new study, the researchers have evaluated how rapidly replanted eelgrass gets populated by various invertebrates. The study has been going on for over two years in a bay near Gåsö island just west of Skaftö in Bohus county, and the findings are very positive. The researchers counted the abundance of invertebrates that live or burrow in bottom sediments or on the surface of bottom sediments. Size less important “The recolonization has been very rapid. After the first three-month growing season, up to 80 percent of the invertebrates had returned to the newly planted eelgrass,” says Eduardo Infantes, marine biologist at the University of Gothenburg. During the summer in 2019, the researchers planted the eelgrass shoots in four test plots of different sizes on the seabed, and with different spacing between the shoots. According to the researchers’ observations in autumn 2020, size has played less of a role in the recovery of biodiversity in the eelgrass meadows. In fact, even if the eelgrass has not had time to grow to the same density as in an established eelgrass meadow, the biodiversity is similar after only two growing seasons as in a reference area of preserved eelgrass in the same bay. Even smaller patches embedded within larger restoration plots showed good results. Their findings were reported in the journal Restoration Ecology. Can save money “This is good news for future restorations and new plantings of eelgrass meadows. We can plant new smaller plots with fewer shoots and this saves money because this is an expensive method for restoring biodiversity on the seabed,” says Eduardo Infantes. Eelgrass meadows have multiple functions that make it imperative to protect them. In addition to their important role in the coastal ecosystem, eelgrass roots bind the sediment and prevent erosion and limit resuspension of sediment in the water. More information: Karine Gagnon et al, Rapid faunal colonization and recovery of biodiversity and functional diversity following eelgrass restoration, Restoration Ecology (2023). DOI: 10.1111/rec.13887